"And now, Mister Bond..."
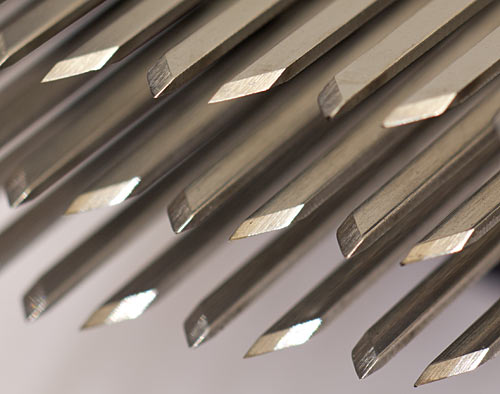
Yes, these are ranks of little pointy blades with angled chisel tips.
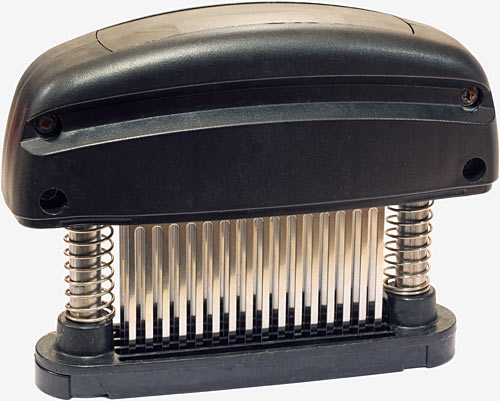
They're all about five centimetres long, and when you use the implement of which they are a part they protrude about 19mm (3/4 of an inch) into some flesh.
Which you later eat.
I don't like cooking. But I can cook a steak. High heat, short time, remember to turn off the smoke detector nearest the kitchen, job done. The less you muck around with it, the better.
(Actually, those annoying scientific-cooking people suggest that frequent turning of a steak is desirable. A religious war will clearly result. The losers get eaten.)
I'm not made of money, though. So the cheaper my steak-meat can be, the better.
I can get a kilo of thick-cut boneless chuck from the local Aldi for eight bucks Australian. That's good for two large steak dinners, or four more reasonable ones. And there's nothing wrong with the flavour of chuck, or what's locally known as "gravy beef" (boned shin), or any number of other cuts from less fashionable parts of the cow. The problem, of course, is that they're full of gristle and connective tissue.
It's surprising how tender even cheap steak can be if you don't overcook it, but the really cheap stuff goes way over the "just needs a lot of chewing" line up into the unacceptable realm where it seems that no amount of chewing is sufficient to actually disintegrate the stuff.
The traditional solution to this problem is, of course, the tenderiser. Which, according to most people, is some kind of mallet, generally resembling a miniaturised version of Kannuki the Giant's signature weapon.
Beating a steak senseless will indeed make it much less chewy, but squashing is not actually a very good way of breaking up gristle; it can take a surprisingly long time, and invariably leaves you with a mutilated beef pancake. That's perfectly acceptable for some dishes, but pulverised beef is pretty close to just being mincemeat ("ground beef", in US parlance). You might as well buy mince in the first place and make meatloaf or rissoles or something, if you ask me.
You can also tenderise meat chemically, with an enzyme or just by letting it go a bit rotten. I haven't tried enzyme tenderisation, but dry-aging my own beef and then shaving off the mould isn't my idea of an appetising activity.
A while ago, though, this Cool Tools post alerted me to the existence of tenderisers that use blades, instead of brute force.
Intrigued by the idea, I tried just laying a cheap steak out on a cutting board and stabbing the hell out of it, all over on both sides, with a couple of little paring knives.
I highly recommend any penny-pinching carnivore try this. It doesn't take very long, and the results are excellent. The meat looks, and cooks, much the same as it did before, but all the stringy stuff has been pre-separated into short pieces. And if you want to marinate your steak in something, the stab-wounds get a lot more flavour into the meat. (I also tried pouring marinade on the steak and then stabbing it, which worked even better but was somewhat messy.)
Satisfied that the technique worked, it was clearly time for me to purchase a kitchen gadget that does the stabbing in a more organised way. The Cool Tools post recommends a "Jaccard SuperTendermatic", with 48 blades in three ranks of 16, which lists on Amazon for $US23.76 ex delivery (cheap to free within the USA, expensive everywhere else), at time of writing.
I'll betcha one of the swish shops in the next suburb over from me has name-brand blade tenderisers too, and I'll also betcha they charge at least a hundred bucks for one.
Instead, I hit eBay and bought a brandless 48-blade unit for a princely $AU17.98 including delivery to Australia, from a Hong Kong eBay seller. That was almost two years ago now; I didn't want to write anything about it before I was sure that the cheap brandless version wouldn't fall apart, maim the user, commit the signature kitchen-gadget failure of being impossible to clean, et cetera.
It doesn't, and I can't imagine that the more expensive brand-name ones work any better.
The current eBay going rate for 48-blade units is less than $AU20 delivered (about $US20 or £13).
(That eBay search doesn't seem to be geo-targeting very well for me here in Australia; here's one that ought to only turn up items that can be shipped here.)
UPDATE: As mentioned in the comments below, there are rotary blade tenderisers as well, that roll like a pizza cutter. Here's a search that I think finds them a bit more effectively than the above searches.
UPDATE 2: Renowned crapvendors DealExtreme also now have 48-blade tenderisers similar to the one I've got. There's a black one and a white one, each for $US17.40 including delivery to anywhere, which I think undercuts the eBay dealers by a little.
It's easy enough to use a blade tenderiser: You just put it on the meat and press down. The blades slide out of slots in a guard on the bottom, and when you release the pressure springs retract the blades again.
The tougher the gristle you're tenderising, the harder it'll grip the blades and resist them retracting. Basically, the more resistance to the blades a given location on the meat has, and the more impressive the crunching sound when you stab it, the more times that area should be stabbed.
The springs are the only weak point of this design, I think. The standard springs in the tenderiser I got were very stiff and heavily pre-loaded, which meant they retracted the blades out of the meat very well, but forced you to push down on top of the meat too hard in the first place, squashing the steak.
I removed the blades and took the handle apart (four simple screws), removed the standard internal springs, and added the natty external coil-over replacements you see in the picture:
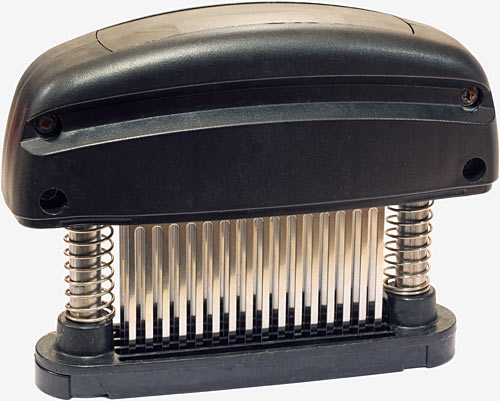
They're shock springs for a model car, and they aren't strong enough to retract the blades by themselves, so I have to push the guard and handle apart a bit myself, but the steak is minimally squashed. I think that's a good trade-off.
The very cheapest blade tenderisers found by that eBay search have only 16 blades, and the spring setup might be better for those. The standard springs don't totally squish the life out of the meat, either; I am unsure how much of my motivation to modify the thing came from an actual need to do so and how much was just my desire to tinker with things.
Apart from that, though, I've had no problems with this thing at all. It works, and it keeps working. I've deliberately bought the toughest cuts of beef I can find - even when they're not actually any cheaper than a slab of chuck - and it's worked, quite quickly, on all of them.
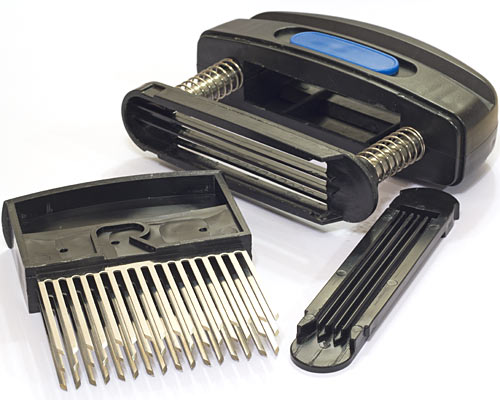
The blade cartridge is removable for cleaning. You push the blue button on the handle to one side and press the tenderiser down on a breadboard, and the blade cartridge pops out the top. The guard at the bottom slides out for cleaning, too. Both of these parts can go in the dishwasher.
Actually, you can put the handle assembly, or the whole assembled tenderiser, in the dishwasher if you like. If you do, though, water will get into the handle, and not want to come out.
The only parts that contact the meat are the blades, the slotted guard and the edge of the guard-holding frame, though, so you can dishwash the removable parts and quickly scrub the frame by hand. As I said in my old review of the AeroPress coffee maker, "impossible to clean" is right up there with "does not actually work" in the list of Mortal Kitchen-Gadget Sins. My blade tenderiser does not have that problem.
Even if you don't have any trouble affording fancy naturally-tender steak, a blade tenderiser could come in handy to make meat more marinatable, or any other time you need a lot of little slits cut in something or someone.
If your grocery budget is tighter, though, one of these things can pay for itself the first time you use it for a family meal. You can even use it after you cook a steak, if there's a gristly bit you missed.
At not much more than twenty bucks delivered for the brand-name one in the States, or for only about twenty bucks delivered on eBay, it comes highly recommended from me.